

The first microfluidic device used for the generation of droplets, a T-junction, was proposed by Thorsen et al. In a stark contrast to the bulk process of shear emulsification that is one of the more illustrative and simple textbook examples of dimensional analysis, generation of droplets in confinement is still not completely understood. Droplet microfluidic systems are also used to create new materials for pharmaceutical 39, 40, 41, cosmetics 42 and food 43 industries.
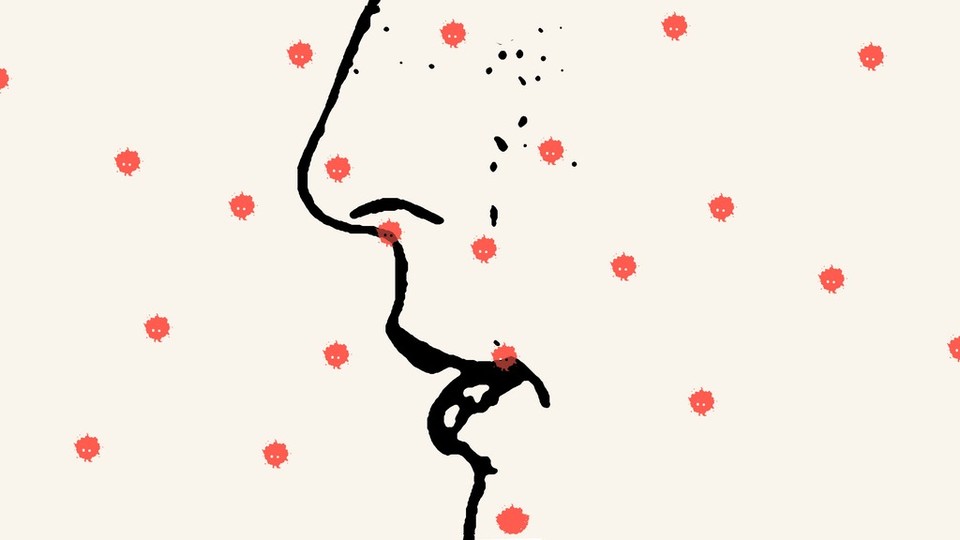
The generation of droplets in microchannels 9, 10 using T-junctions 11, 12, 13, 14, 15, 16, 17, 18, 19, flow-focusing 20, 21, 22, co-flow 23, step emulsification 24, 25, 26, 27, 28, 29 and parallel devices 30, 31, 32, opened the new discipline of droplet microfluidics 33, 34 that revolutionized analytical methods in biology 35 and medicine with digital assays 36, single cell sequencing 37, or systems for research on biological evolution 38.
#Droplet transition principle app full#
A striking example is the flow of a single droplet through a microchannel 5, 6, 7, 8 for which the most basic question how the speed of the droplet depends on flow conditions, fluid properties, and the level of confinement still lacks a full answer. In spite of the beautiful regularity in flows of droplets in microfluidic networks at low Reynolds and capillary numbers, their dynamics offers rich phenomenological complexity 1, 2, 3, 4 that prohibits predictive understanding. Our model predicts droplet size and characterizes the transitions from leaking into squeezing and from squeezing into jetting, unifying the description for confined droplet generation, and offering a practical guide for applications.

For the first time we formally account for the flow around forming droplets, to predict and discover experimentally an additional regime-leaking. Here, we reveal that further reduction of-already negligibly small-shear unexpectedly re-introduces the dependence of droplet size on shear/capillary-force ratio. The size of confined droplets is controlled by the ratio between shear and capillary forces when both are of the same order, in a regime known as jetting, while being surprisingly insensitive to this ratio when shear is orders of magnitude smaller than capillary forces, in a regime known as squeezing. While shear emulsification is a well understood industrial process, geometrical confinement in microfluidic systems introduces fascinating complexity, so far prohibiting complete understanding of droplet formation.
